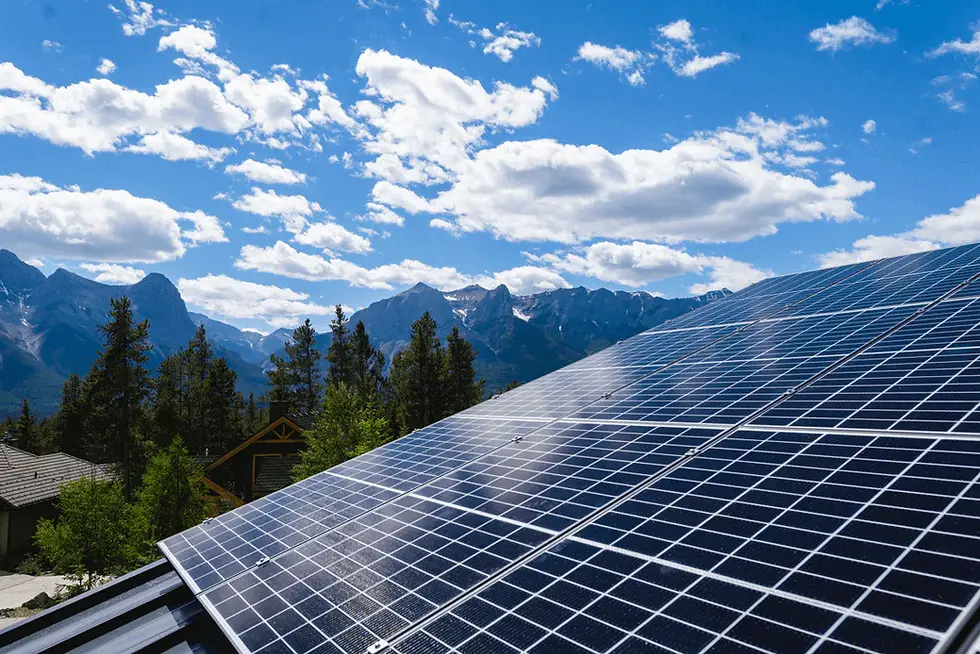
A photo showing a bunch of solar panels attached to the roof of a house.
Solar panels are devices that turn sunlight into electricity. From rooftops to big fields to even on small gadgets, we can find them almost anywhere around us, and their primary purpose is to power things without burning fuel. They’re part of a push to find cleaner ways to make energy, since sunlight is free and doesn’t release any harmful smoke or gases. To truly understand how they work, we need to look at the parts inside and what happens when sunlight hits them.
The Parts of a Solar Panel
A solar panel starts with small units called solar cells, which are the heart of the whole setup, and they are where sunlight gets turned into electricity. Each cell is made from a material called silicon, which comes from sand and gets purified into a crystal form. Silicon has properties that let it handle electrons, which, if you don’t know already, are tiny particles that carry electric charge. The cells are thin squares, usually about six inches on each side, and they’re arranged in rows on the panel. A typical panel might have 60 or 72 cells, depending on its size.
Each solar cell has two layers of silicon stacked together. One layer gets treated with a chemical like phosphorus, which adds extra electrons to it. This is called the n-type layer because it has a negative charge from those electrons. The second layer, on the other hand, gets coated with something like boron, which leaves it short on electrons and gives it a positive charge. That’s the p-type layer. When these two layers press against each other, they form a boundary called the p-n junction, which is where the action happens (but we’ll get to that later).
On top of the cell, there’s a thin grid of metal lines. These lines are contacts that collect the electricity once it’s made, and they’re usually silver or another metal that conducts well. Underneath, a solid metal sheet covers the back to complete the circuit. Glass protects the front of the panel, letting sunlight through while keeping out rain or dust, while a plastic layer seals the back. In addition, there is also a frame that is responsible for holding everything together. Wires connect the cells to each other and to the outside world, carrying the power away. All of these parts work as a team, but the real process starts with the silicon and sunlight.
How Sunlight Turns into Electricity
The whole process begins when sunlight hits the solar cell. Sunlight is made of tiny packets of energy called photons, and when these photons hit the silicon, they knock electrons loose from their spots in the atoms. Silicon atoms normally hold their electrons tight, but these photons have so much energy that the electrons are broken free. This happens all over the cell, but it’s not random chaos. The p-n junction organizes everything.
At the junction, as mentioned previously, the n-type layer has extra electrons from the phosphorus, while the p-type layer has spaces where electrons could fit (because of the boron). When a photon knocks an electron loose, the junction pulls it toward the n-type side. The empty space left behind, called a hole, drifts toward the p-type side. Electrons and holes want to balance out, but the junction acts like a wall that keeps them apart. This separation builds up a difference in charge, with negative on the n-type side and positive on the p-type side. That difference is what we call voltage.
Voltage alone doesn’t make electricity flow. The metal contacts on the cell connect to a circuit, like wires hooked to a battery or a house’s power system. When the circuit closes, electrons start moving from the n-type side, through the wires, to the p-type side, trying to fill those holes. This movement is called electric current. Together, the voltage and current create power, measured in watts. One photon might free one electron, but when billions of photons hit the cell every second, the current adds up fast. Simply put, sunlight shakes electrons loose, and the p-n junction turns that mess into a steady flow of electricity.
Turning Direct Current into Usable Power
Solar cells produce electricity in a form called direct current, or DC. In DC, electrons flow in one direction, like water down a straight pipe. Most homes and machines, though, use alternating current, or AC, where the flow switches back and forth many times a second. To fix this, solar panels connect to a device called an inverter. The inverter takes the DC from the cells and flips it into AC that matches what the power grid or a house needs.
The inverter does its job by using switches and circuits inside it. It chops the DC into pulses and then smooths those pulses into a wave that mimics AC. This wave has to line up with the grid’s frequency, usually 60 cycles per second in the United States. If the panel isn’t tied to the grid—like in a cabin or a boat—the inverter still makes AC for appliances, or sometimes the system stays DC for things like batteries. Either way, the inverter is key because raw solar power wouldn’t work for most of what we plug in.
Wires carry the electricity from the inverter to wherever it’s needed. In a house, it might go to outlets or lights. In a bigger setup, it feeds into the grid, where power companies distribute it. Panels often link together in strings, boosting the total voltage and current to handle larger loads. The system adjusts based on how much sunlight hits the cells, with more light meaning more electrons, which leads to more power. At night or in clouds, output drops, so some setups use batteries to store extra juice for later.
What Affects How Well It Works
Not every photon turns into electricity. Some bounce off the glass or the cell’s surface. Others pass through without hitting anything useful. Scientists call this efficiency, and it measures how much of the sunlight’s energy becomes electric power. Most solar cells today convert about 15 to 20 percent of the sunlight they get. The rest turns into heat or just doesn’t get used. Better materials and designs push that number up, but there’s always a limit because of how silicon and light interact.
Temperature messes with things too. Hot cells don’t work as well because heat makes electrons move less predictably. That’s why panels in deserts might underperform compared to cooler spots, even with more sun. Dust or dirt on the glass blocks light, so people clean panels to keep them running right. The angle matters too because panels facing the sun straight on catch more photons than ones tilted away. In big solar farms, machines tilt the panels to follow the sun all day.
The quality of the silicon counts. Pure crystals work better than cheaper, messier ones. Making pure silicon takes energy and money, so there’s a trade-off between cost and performance. Damage to the cells, like cracks from hail, cuts output by breaking the circuit. Over time, cells wear out, losing a bit of efficiency each year. A good panel might last 25 or 30 years, but it won’t pump out as much power at the end as it did at the start.