What Exactly Is Antimatter, And What Is It Used For?
- Krishna Rathuryan
- Jan 22
- 3 min read
Updated: Jan 23
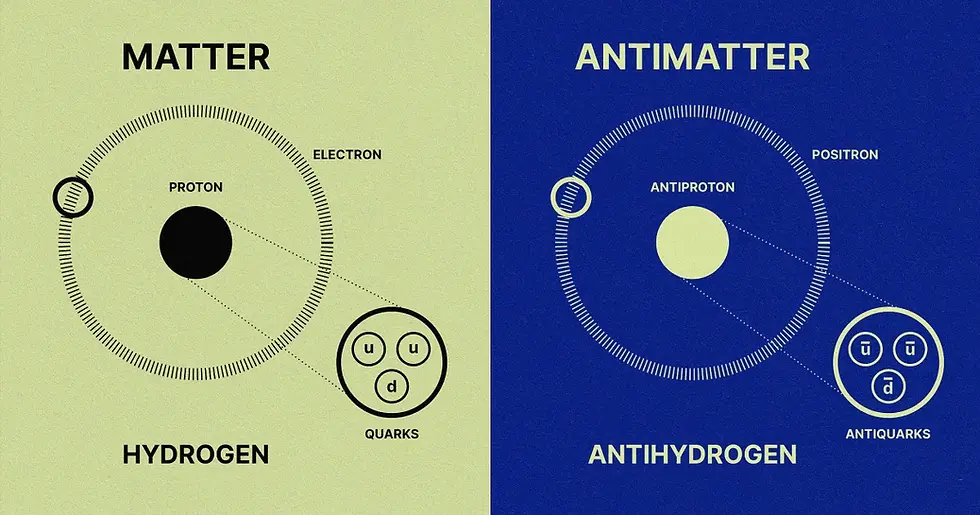
A graphic showing the difference between Hydrogen and Antihydrogen, Hydrogen’s antimatter counterpart.
Antimatter is, in a short phrase, the opposite of matter. If you think of everything that is around you—chairs, tables, or even air—that is all regular matter. But for every variety of matter particle, there is an antimatter particle that opposes it. For example, the most common particle of regular matter, the electron, has an antiparticle known as the positron.
Antimatter particles have the same mass as their corresponding matter particles but carry opposite charges. If an electron carries a negative charge, then a positron carries a positive one. When matter and antimatter come into contact, they absolutely annihilate each other, transforming their mass into energy. This process is described by Einstein's famous equation E = mc^2, where E is energy, m is mass, and c is the speed of light.
How Did We Discover Antimatter?
The concept of antimatter first came from a British physicist named Paul Dirac in 1928 when he was working on a theory to describe how electrons move at speeds close to the speed of light. His equations suggested there should be particles with the same mass as electrons but opposite charge. It was not until 1932 that this prediction was verified when Carl Anderson discovered evidence for positrons in cosmic radiation by using a device called the cloud chamber, which made the tracks of particles visible. Since then, other antimatter particles related to protons and neutrons have been identified, including antiprotons and antineutrons.
Where Does Antimatter Come From?
Antimatter is not something you can just stumble upon in nature. The universe seems to be made up mostly of matter, with very little antimatter. Scientists believe that the Big Bang produced equal amounts of matter and antimatter, but for reasons not fully understood, only matter ended up dominating.
Nowadays, antimatter production largely comes from experiments where atomic or particle collisions occur. In places like CERN—the European Organization for Nuclear Research— protons are accelerated nearly to the speed of light, and when they collide, they can generate matter-antimatter pairs.
Antimatter in Science and Medicine
More importantly, antimatter is not just a curiosity; it also has real-world applications. In medicine, positrons are used in a technique called Positron Emission Tomography (PET). Here, patients receive a radioactive substance containing positrons. These positrons interact with electrons in the body, annihilate, and produce gamma rays, which are then detected to show the activity of metabolic processes.
Antimatter also helps physicists study basic questions about the universe. Why is there more matter than antimatter? Are there asymmetries in the laws that determine the behavior of particles and antiparticles? These questions are part of particle physics studies.
Problems with Antimatter
Handling antimatter is incredibly challenging though. If you put antimatter in a container made of regular matter, it would annihilate instantly. Scientists use magnetic fields to trap antimatter particles, but even then, storage is temporary because eventually, the particles will touch the matter of the trap or each other and annihilate.
Moreover, creating antimatter is highly energy-consuming and expensive. Producing just a gram of antimatter would require energy costing 62.5 trillion dollars, making it one of the most, if not the most, expensive materials ever made.
The Future of Antimatter Research
Research into antimatter continues with the aim of understanding its properties better, improving production methods, and finding more applications. The main goal is not just practical use but also to solve some profound mysteries of physics.
Scientists hope that ongoing experiments will yield answers. Projects like the Alpha experiment at CERN, where researchers trap antihydrogen (the antimatter counterpart to hydrogen), are steps toward understanding how antimatter behaves under different conditions.
Conclusion
Antimatter, though rare and exotic, is very important to our understanding of the universe. From its theoretical prediction to its use in medicine and beyond, antimatter challenges our basic questions about the nature of existence. It's an area where science fiction often meets hard, exacting science. As scientists do more research, each discovery about antimatter will improve our knowledge and fuel our curiosity about the universe.