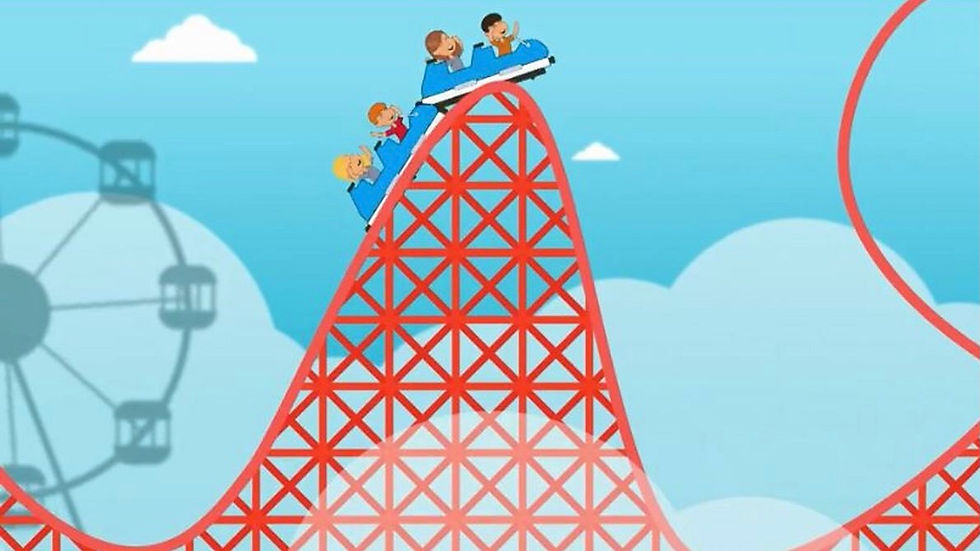
A roller coaster car with maximum potential energy.
Energy, in general, allows us to explain how things move, change, or remain still in the world around us. Originally, the definitions and kinds of energy included kinetic and potential energy. These two types of energy can be connected with the behavior of objects both when they are in motion and at rest. To understand them, we must first know what energy is, how it works, and how kinetic and potential energy differ in their definitions, calculations, and real-world examples.
What Is Energy?
Before one can explore kinetic and potential energy, it’s important to know what energy is in the context of science. Energy is the ability to do work, which means it allows objects to move, lift, or change in some way. We don’t see energy directly; rather, we only perceive its effects. For example, with a ball descending a hill, energy is responsible for that descent. A book lying on a shelf represents stored and potentially usable energy. Energy is measured in joules, and it follows an important rule in science: energy cannot be created nor destroyed; it can only be transferred or changed from one form to another. The name of this famous law is "the law of conservation of energy."
Defining Kinetic Energy
Kinetic energy is the energy an object has because it is moving. A vehicle moving on the road, a person running, a river flowing, or any object in motion is said to have kinetic energy. The kinetic energy of something depends on two factors: its mass and the speed of its motion. An object with greater mass has more kinetic energy than a lighter object, assuming both are travelling at the same speed. Similarly, when two objects are identical except for speed, the faster-moving object has more kinetic energy than the slower object.
Scientists have a formula for the calculation of kinetic energy. Kinetic energy equals one-half mass times velocity squared; in symbols, it is written as KE = 0.5(m)(v^2). In this equation, "m" means mass, measured in kilograms; "v" means velocity or speed, measured in meters per second; "KE." means kinetic energy and is always measured in joules.
Here’s a more specific example with real numbers: a 2 kg ball rolls at a speed of 3 m/s. What is its kinetic energy?
KE= 0.5 × 2 × 3^2 = 0.5 × 2 × 9 = 9 joules
The formula establishes that speed has more influence over kinetic energy than mass because speed is squared while mass is plugged in just as it is. Thus, doubling the speed makes the kinetic energy increase four times, not two.
Kinetic energy is present in many situations. When a bicycle moves, the wheels, frame, and rider all have kinetic energy because they are in motion. When a hammer strikes a nail, the hammer’s kinetic energy is transferred to the nail, driving it into the wood. Even tiny particles, like air molecules, have kinetic energy as they move around. In all these cases, the energy of motion is what defines kinetic energy.
Defining Potential Energy
Potential energy is the energy possessed by an object solely based on its position or status. This type of energy doesn't involve motion. Instead, it’s stored energy, ready to be released or converted into other forms. By far, the most common form of potential energy is gravitational potential energy, which depends on the object's height above the ground and its mass. An object higher in the air possesses more potential energy simply because it has more time fall and acquire kinetic energy. Similarly, if an object and another are at the same height, the heavier one has more potential energy.
To calculate gravitational potential energy, scientists use another formula. The formula is potential energy equals mass times gravity times height. In symbols, it is written as PE = m × g × h. Here, “m” stands for mass, measured in kilograms, “g” stands for the acceleration due to gravity, which is about 9.8 meters per second squared on Earth, and “h” stands for height, measured in meters. The result, potential energy, is measured in joules. For example, if a 5-kilogram book is on a shelf 2 meters above the ground, its potential energy is calculated as follows: 5 times 9.8 times 2, which equals 98 joules. This formula shows that potential energy increases with both mass and height. If the height doubles, the potential energy doubles. If the mass doubles, the potential energy doubles as well.
Potential energy is not limited to gravity; elastic potential energy is another type, and it is energy that is stored in objects, like rubber bands or springs, that can be stretched or compressed. A rubber band held in a stretched condition has potential energy, and when released, that energy is converted to kinetic energy.
Comparing Kinetic and Potential Energy
Kinetic and potential energy are closely related because they often transform into each other. When an object falls, its potential energy decreases as its height decreases, but its kinetic energy increases as its speed increases. At the top of its path, a falling object has maximum potential energy and zero kinetic energy if it is not moving. At the bottom, just before it hits the ground, it has maximum kinetic energy and zero potential energy, assuming the ground is the reference point for height. The total energy, which is the sum of kinetic and potential energy, stays constant if no other forces, like air resistance, interfere. This balance is a direct result of the law of conservation of energy.
Consider a pendulum swinging back and forth. At the highest point of its swing, the pendulum has maximum potential energy because it is farthest from the ground. At this moment, its speed is zero, so its kinetic energy is zero. As the pendulum swings downward, its height decreases, reducing its potential energy, but its speed increases, raising its kinetic energy. At the lowest point of the swing, the pendulum has maximum kinetic energy and minimum potential energy. As it swings back up, the process reverses, with kinetic energy turning back into potential energy. This constant exchange between kinetic and potential energy continues, though in real life, some energy is lost to air resistance and friction, causing the pendulum to eventually stop.